UT Research Holds Promise for Medical, Scientific Breakthroughs
Highlights
- The creation of “smart” biomaterials is within reach
- Those materials could help restore movement to patients with immobility issues
- The materials also have the ability to learn, which holds promise for unmanned search and rescue operations
Neuromorphic computing came about in the last few decades as way to mimic how synapses and neurons in the brain process, learn, and remember information. With the potential to create more capable and efficient computing systems, this approach seeks to integrate concepts from physics, computer science, mathematics, biology, and electrical engineering.
More recently, methods for assembling and characterizing membranes have been developed that allow researchers to craft mimics of living cells with engineerable transport properties.
Combining the two concepts to create “neuromorphic cell mimics” could also lead to “smart” bio-materials with applications ranging from drug delivery to unmanned rescue missions.
Interim Engineering Dean and John Fisher Distinguished Professor Mark Dean, Professor James Plank, and Associate Professor Garrett Rose of the Min H. Kao Department of Electrical Engineering and Computer Science, and Assistant Professor Andy Sarles of the Department of Mechanical, Aerospace, and Biomedical Engineering have joined forces with ORNL’s Pat Collier to figure it out.
Dean, Plank, and Rose bring a vast knowledge of neuromorphic circuits and computation to the team, while Sarles and Collier have built their expertise around understanding biomembranes.
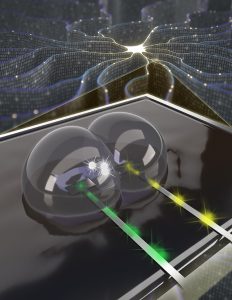
Andrew Sproles, ORNL
“For our work, we’re using the membranes that Andy works with to stand in for the neural synapses that we normally simulate,” Rose said. “Sending an electric charge through the membrane makes the membrane react, and varying that charge makes it react differently.”
Although similar experiments have been done before using solid-state materials, the team’s use of soft, biomolecular ‘memristors’—variable resistors that “remember” state changes after being powered off and then back on again—sets their research apart. Memristors can also operate with multiple levels of input rather than simple on-or-off binary switches, making them ideal to help the team replicate complex neural synapses.
“Memristors based on the inherent functions of biological molecules have basically allowed for the creation of tunable devices for information processing in materials and products,” Sarles said. “Their reactions vary based upon new stimuli and what they ‘remember’ from before.”
And that is the true cornerstone of the research. Utilizing memristors in tunable cell mimics has made it possible to “train” such materials to compute, learn, and remember.
By connecting them to circuits, the team hopes to simulate different scenarios and reactions from the cell mimics and eventually design them to enable autonomous control of other systems, such as directing repetitive, memory-based tasks like moving limbs, stopping neuro skeletal disorders, or treating other afflictions.
Plank said that the first big step in creating neuromorphic cell mimics is to simulate as many outcomes as they can and then use those in a realistic scenario to inform the next round of simulations.
“Right now, we’re at a stage where we’re using about 30 to 40 of Andy’s cell mimics to make a processor,” Plank said. “Since those cells resemble droplets, we’ve basically got a bubble-based processor.”
In addition to medical applications, networks of neuromorphic cell mimics can learn to follow patterns, for instance, which could help teach unmanned vehicles how to conduct search and rescue missions, or help drone deliveries.
So, while their physical sizes might be small, the roles that they could play are huge.